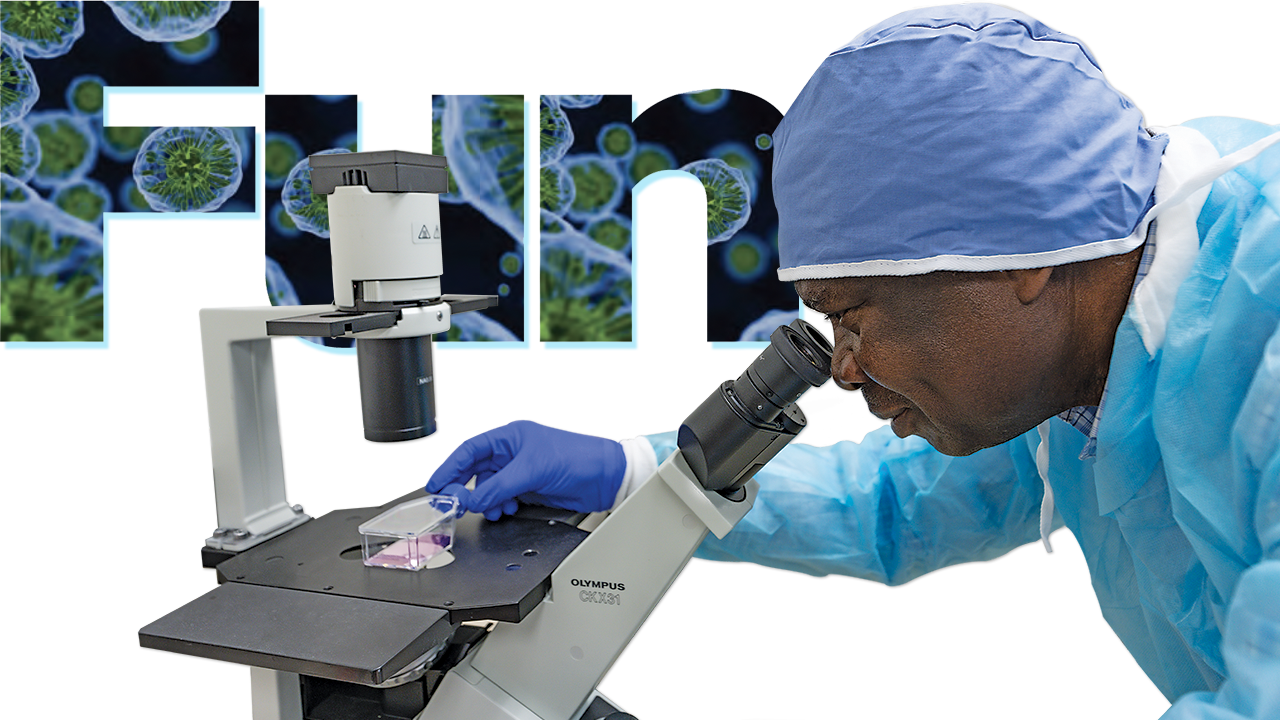
Fundamental Research Drives Discovery
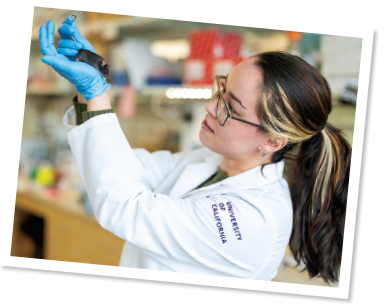
Depending on your generational definition of the word, “basic” science could conjure a very different understanding. Contrary to the modern interpretation, basic is anything but unoriginal or unexceptional. This type of scientific inquiry forms the essential foundation or starting point for fundamental discoveries and innovation.
Our school’s reputation as #1 in the nation is built largely on the strength of our basic research endeavors. Those efforts may not immediately cure cancer or prevent Alzheimer’s, but they form the foundation for future therapeutics that could.
A common challenge for fundamental researchers is to obtain funding for scientific inquiries that don’t often offer an obvious or immediate benefit—especially to human health. It may take decades, but each new discovery provides a critical building block to illuminate a new and unexpected research direction until researchers arrive at a place where they can see a benefit.
While federal support and other sources for fundamental research has steadily decreased in the last decade, the UC Davis School of Veterinary Medicine consistently leads veterinary schools around the nation with its robust research funding. In the last fiscal year, the school has been awarded $70 million toward research projects.
The fun part of fundamental science is being able to answer the questions motivated by a gap in knowledge. We get to ask, why is this important, and then collaborate with others to figure it out.”
—Professor Danika Bannasch
“Key scientific advancements would be impossible without the basic understanding of biological processes,” said Professor Danika Bannasch, associate dean of research and graduate education. “The fun part of fundamental science is being able to answer the questions motivated by a gap in knowledge. We get to ask, why is this important, and then collaborate with others to figure it out.”
This article explains the significance of six recent fundamental research studies and their implications for improving human, animal and environmental health.
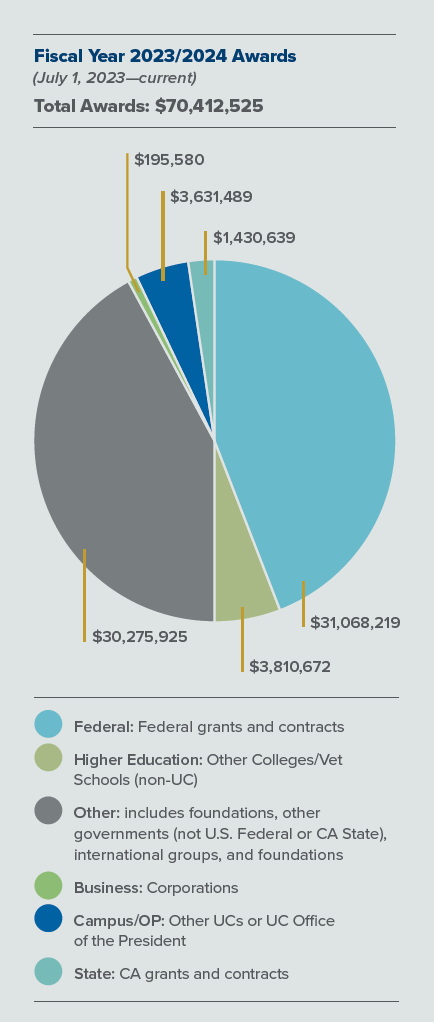
The UC Davis School of Veterinary Medicine consistently leads veterinary schools around the nation with its robust research funding. In the last fiscal year, the school has been awarded $70 million toward research projects.
1. Common Chemicals Pose Harm
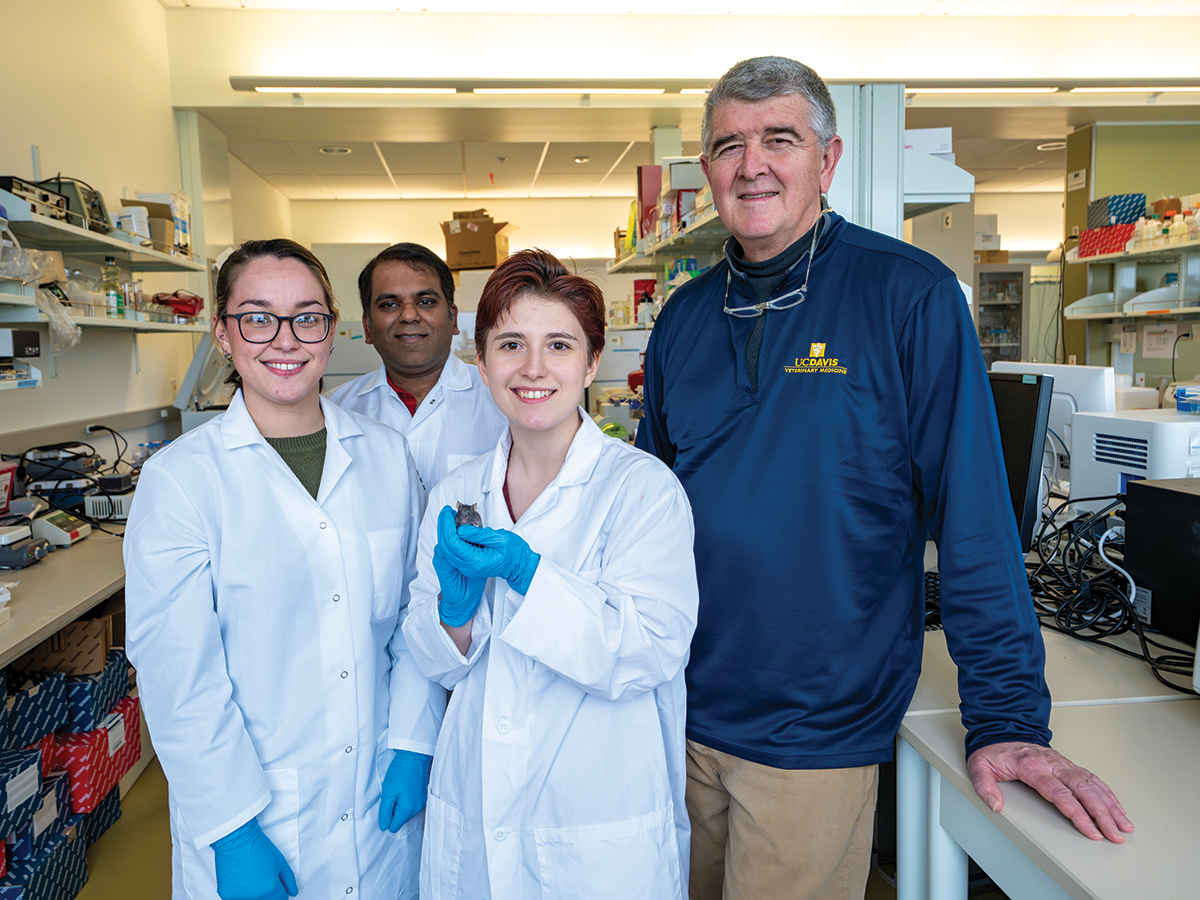
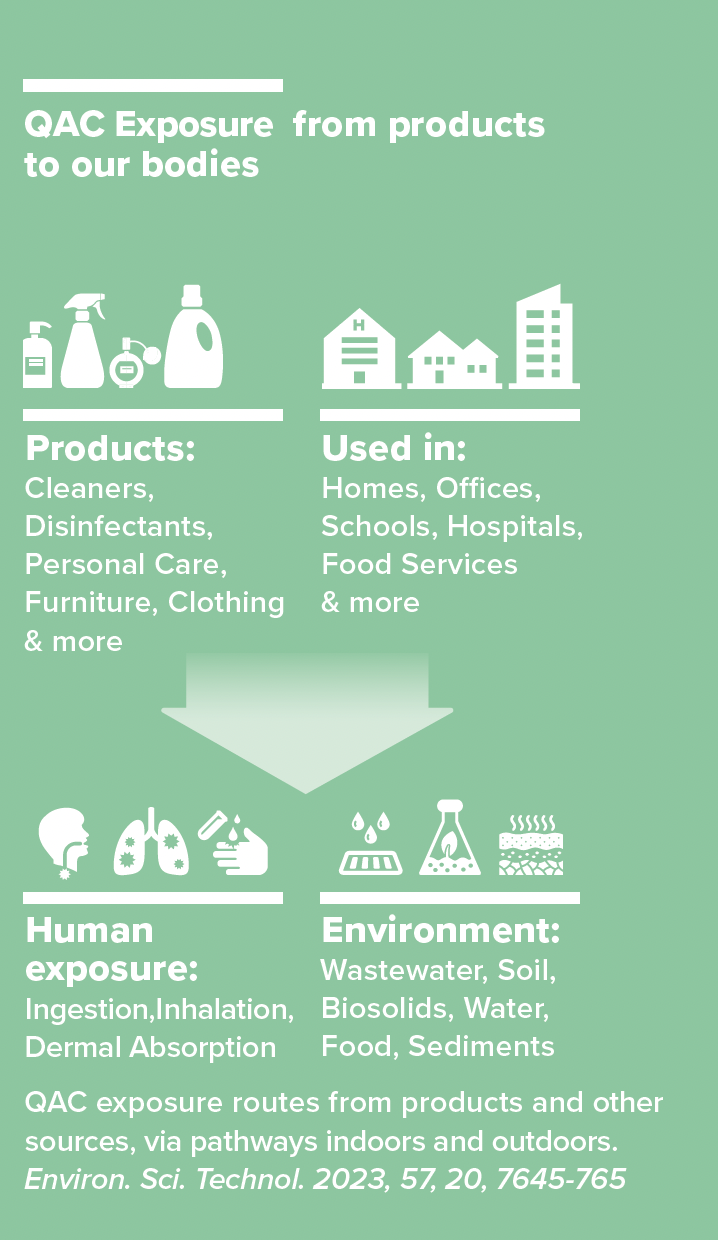
Professor Gino Cortopassi and colleagues have been sounding the alarm about ubiquitous quaternary ammonium compounds (QACs) since 2017. This chemical class has been used for decades in antimicrobials, preservatives, antistatic agents and for other functions in cleaning, disinfecting, personal care products, and durable consumer goods.
Their initial in vitro study published in Environmental Health Perspectives indicated that QACs inhibited mitochondria—the powerhouses of the cell—as well as estrogenic functions in cells. Subsequent studies by Virginia Tech colleagues demonstrated a link between QACs and neural tube birth defects in both mice and rats. A 2021 collaborative study was the first to establish that QACs were able to pass from environmental exposure into human blood.
“That was shocking,” Cortopassi said. “QACs have been used as disinfectants since the 1940s and the dogma was that these chemicals didn’t pass into the bloodstream. We were able to show QACs did make it into human blood, and also showed significant correlations between blood QAC levels and blood inflammation markers and mitochondrial function.”
QAC use accelerated in response to the COVID-19 pandemic; it also increased after triclosan and other antimicrobials were banned by the U.S. FDA in 2016. Triclosan's ban was driven by UC Davis Professor Emeritus Isaac Pessah’s research finding that this disinfectant caused cardiac arrythmias in mice. Most recently, a multidisciplinary, multiinstitutional team of academic authors (including Cortopassi and other UC Davis researchers), governmental, and nonprofit organizations published a Critical Review in Environmental Science and Technology. Their goal is to highlight the emerging information on adverse human, animal and environmental health impacts of QACs. Negative ecological effects include acute and chronic toxicity to susceptible aquatic organisms. Suspected or known adverse health outcomes for humans and animals include skin and respiratory effects, developmental and reproductive toxicity, disruption of metabolic function such as lipid homeostasis, and impairment of mitochondrial function.
QACs have been used as disinfectants since the 1940s and the dogma was that these chemicals didn’t pass into the bloodstream. We were able to show QACs did make it into human blood, and also showed significant correlations between blood QAC levels and blood inflammation markers and mitochondrial function.”
—Professor Gino Cortopassi
Thanks to a National Institutes of Health Exploratory Development Research Award, Cortopassi’s lab is now tackling the question of how QACs enter the bloodstream. Current studies underway provide preliminary indication of a 200-fold increase in toxicity if QACs are delivered as aerosol versus orally in a mouse model. This is of particular concern as spray cleaners containing QACs are used everywhere —including hospitals and veterinary clinics.
Cortopassi explained that his inquiry into this field began when he started looking for molecules that boost mitochondrial function several decades ago. His first published study in 1990 in this area showed that mitochondria degrade with age, so he began screening thousands of molecules looking for those that may boost function. What he found instead were things in the environment that damaged mitochondria and inhibited function. Ah, this is unique, he thought. Let’s look into it more deeply.
So, he followed a different path than he originally set out on—as many scientists do when their initial assumptions are challenged or a fresh line of inquiry emerges. And each new study builds on the foundation of a previous discovery.
“With this comprehensive review, we’re trying to raise people’s consciousness that QACs could be toxic and causing harm,” Cortopassi said. “We have to keep doing the science to show whether they pose a real health threat to people, animals and the environment.” —By Trina Wood
2. New Promise for Treating Glioblastomas
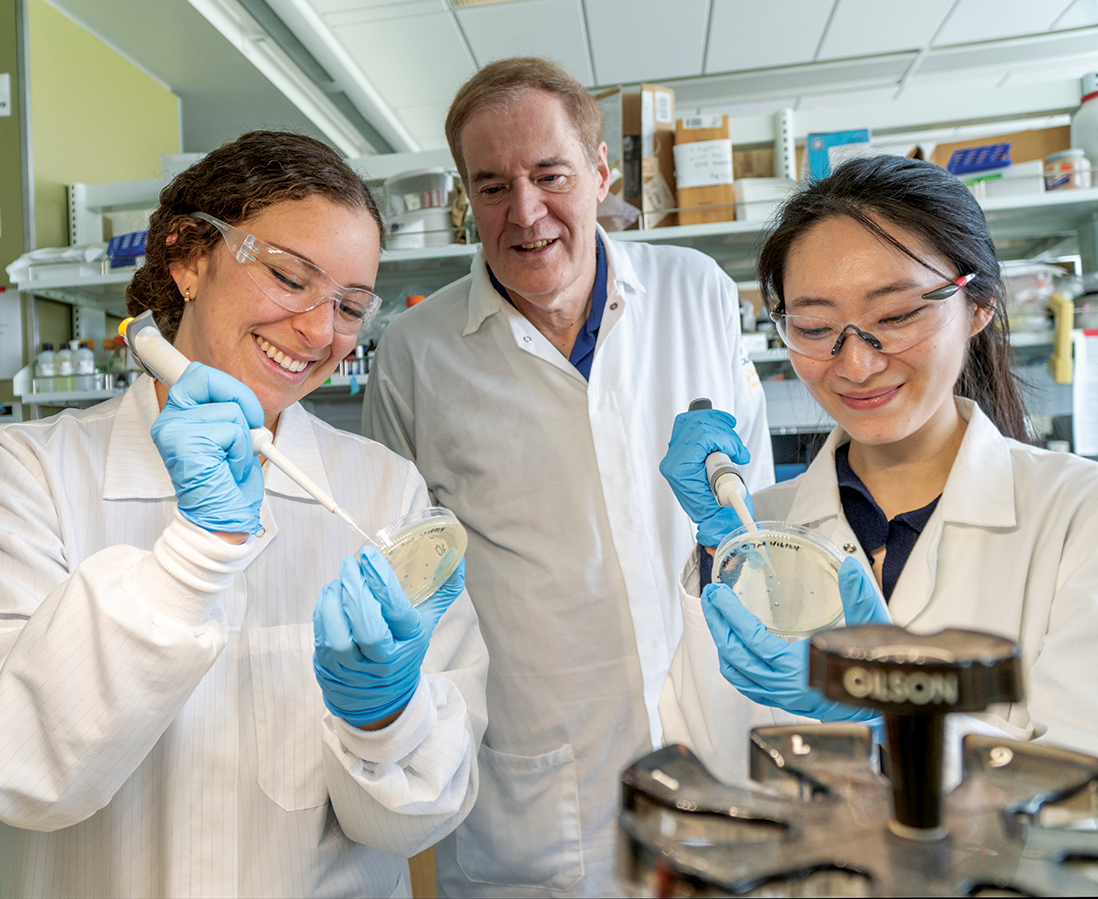
In newly diagnosed glioblastoma (GBM) patients, the researchers saw 83% disease control rate, where the disease shrinks or remains stable over a certain time period. For patients with recurrent GBM tumors, 67% experienced disease control.
Glioblastoma multiforme (GBM) is the most common and malignant form of brain cancer and is associated with high morbidity and mortality. Despite recent advances in the detection and treatment of these tumors, the 5-year survival rate remains below 5%, substantially lower than most other solid tumor types.
That survival rate may soon rise, thanks to a promising new therapeutic developed as a result of fundamental research by Associate Professor Jim Angelastro and colleagues. A recent review in the journal Cells discusses the identification of three oncogenic, or tumor related, transcription factors as targets for treating brain and other cancers; the strategies designed with those factors to suppress cancer cell growth; and the drug development to implement the strategies.
The Cells review focuses on transcription factors ATF5, CEBPB and CEBPD. Transcription factors are proteins that help to regulate genes. In this case, glioblastoma cells rely on these transcription factors for their survival. If these factors can be turned off, cancerous cell growth can be suppressed. The trick is to find a way to penetrate cancer cells to disable these factors and promote cell death.
Angelastro began his work with one of these transcription factors, known as ATF5, while he was still a neurobiology postdoctoral student at Columbia University with Dr. Lloyd Greene (a co-author on the Cells paper). After his uncle died from a recurrent glioblastoma, Angelastro focused his research on which genes played a role in cancer formation with the idea that if he could find a way to turn them on/off, he could selectively suppress the growth and survival of tumor cells. Their research led to patents at Columbia/University of California for ATF5 to be used in future therapeutic development.
After Angelastro joined the faculty at the UC Davis veterinary school, he further developed a transgenic mouse model with a dominant negative ATF5 transgene. Dominantnegative describes a type of genetic mutation that results in a protein that interferes with the normal function of the wild-type protein. In other words, the mutant protein “dominates” the normal protein, preventing it from functioning correctly. Working with Charles Cates DVM ’11 (then a student in the Students Training in Advanced Research program), Angelastro developed a cellpenetrating dominant negative ATF5 peptide and showed similar results compared to the transgene in his mouse tumor model; the dominant negative ATF5 peptide killed off cancerous tumors within eight days and these tumors never reoccurred.
As evidence grew for the role of ATF5 in developing new cancer drugs, Columbia licensed the technology to Sapience Therapeutics, a start-up launched in 2016. The company developed ST101, a variant of the cell-penetrating dominant negative ATF5 peptide drug based on the precursor peptide that Angelastro had developed, and launched human clinical trials. After showing the treatment was safe in Phase I, they entered Phase II in July 2021 with glioblastoma, melanoma, and types of prostate and breast cancers.
The results presented at the Society for Neuro-Oncology last November were highly encouraging. In newly diagnosed GBM patients, the researchers saw 83% disease control rate, where the disease shrinks or remains stable over a certain time period. For patients with recurrent GBM tumors, 67% experienced disease control. More importantly, patients who achieved disease control have resumed “normal” lives.
“Of course, I would like to see 100% disease control, but nothing in life is 100%,” Angelastro said. “This new therapeutic doesn’t save lives, but it enhances and extends lives. And it gives us a target to develop further treatments for other types of cancers. I’m pretty happy where it’s going.” —By Trina Wood
3. Controlling Type-2 Diabetes
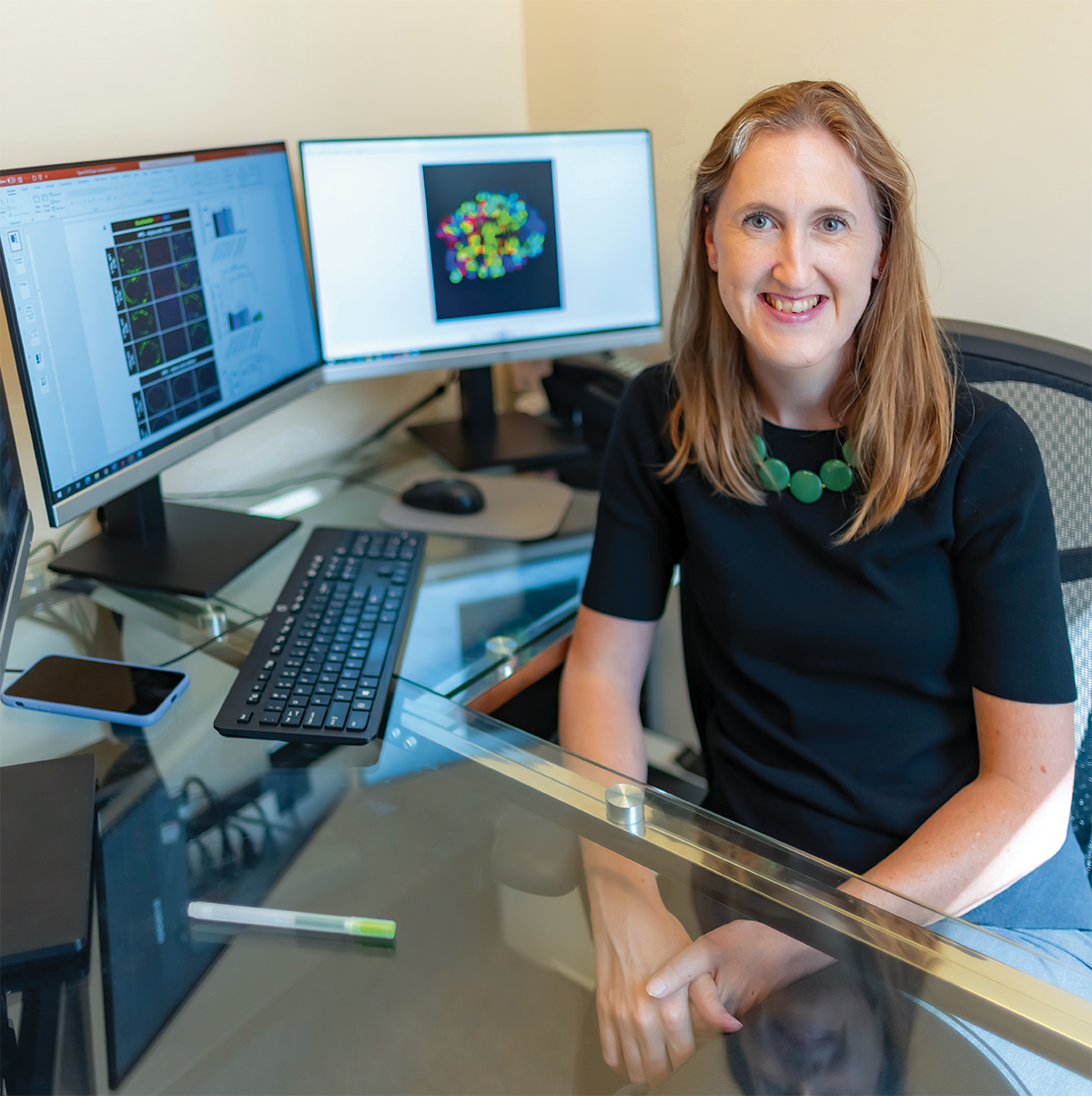
Professor Bethany Cummings’s research helps to understand how current GLP-1-based diabetes drugs work and may bring scientists closer to developing more effective drugs that stimulate the body’s own production of GLP-1 without the side effects of common diabetes therapeutics.
Type-2 diabetes is a common disease caused by an excess of blood glucose that can lead to severe health problems. New diabetes medications like liraglutide (Victoza) and semaglutide (Ozempic) have become popular for promoting weight loss and improving blood sugar levels. These drugs stimulate the glucagon-like peptide-1 receptor (GLP-1R) to lower blood sugar levels by increasing pancreatic production of insulin.
A new report in Science Advances by Professor Bethany P. Cummings and DVM/Ph.D. trainee Dr. Marlena Holter expands our understanding of how these drugs work and could lead to better therapeutics for control of type-2 diabetes.
Scientists have long thought that the hormone GLP-1—which activates the GLP-1 receptor—was primarily produced in the gut. However, this study indicates that GLP-1 production relies on more complicated communication between cells in the pancreas, not just the gut.
Hormones that control blood sugar levels in the body are produced by clusters of pancreatic cells called islets, which contain various cell types that are defined by the hormone that they produce. Islet alpha cells produce glucagon, a hormone that elevates blood sugar levels by stimulating the liver to release glucose. Excess glucagon production is one of the causes of type-2 diabetes.
Glucagon’s polar opposite is insulin, produced in islet beta cells. Insulin deposits blood sugar into cells for energy utilization or for storage. GLP-1 enhances insulin secretion after a meal. With type-2 diabetes, beta cells don’t produce enough insulin to counteract glucagon.
Liraglutide and other medications target beta cells to increase insulin secretion. However, recent diabetes research compelled Cummings to take a closer look and challenge entrenched notions of pancreatic islet functions.
“I was fascinated by clinical research reporting that bariatric surgery causes remarkably high rates of type-2 diabetes remission,” Cummings said. “It’s not just due to weight loss; some people experience remission immediately after surgery.”
She developed rodent models of bariatric surgery to understand how the process works and found that GLP-1 production dramatically increases after surgery in the least likely of places—alpha cells that typically produce glucagon and advance type-2 diabetes.
These findings led to her current research focus: discovering how pancreatic alpha cells modulate the effects of liraglutide. The medication works, in part, by stimulating insulin secretion and inhibiting glucagon secretion. Cummings and her research team worked to understand how alpha and beta cells communicate with one another to mediate the effects of liraglutide.
“What we’re seeing is that, if you increase the signaling of GLP-1 receptors on beta cells, this stimulates the alpha cells to change their hormone production to produce GLP-1,” she explained. “The alpha cells then contribute to liraglutide’s efficacy in stimulating insulin secretion.”
The team also sought the origins of the beta cell’s ability to control alpha cell functioning. They found that liraglutide treatment of beta cells significantly downregulates the signaling protein 14-3-3-zeta, which regulates liraglutide’s effects. The team’s next step is identifying the secreted factor that beta cells use to prompt alpha cells to alter hormone production, using the signaling protein 14-3-3-zeta as a key.
Although this work took place in mouse models, it was validated in human islet models to confirm the presence of a shared hormonal pathway.
Cummings’s research helps to understand how current GLP-1-based diabetes drugs work and may bring scientists closer to developing more effective drugs that stimulate the body’s own production of GLP-1 without the side effects of liraglutide and other diabetes therapeutics. —By Jen DeMoss
4. Common Environmental Gas Causes Neurodegeneration
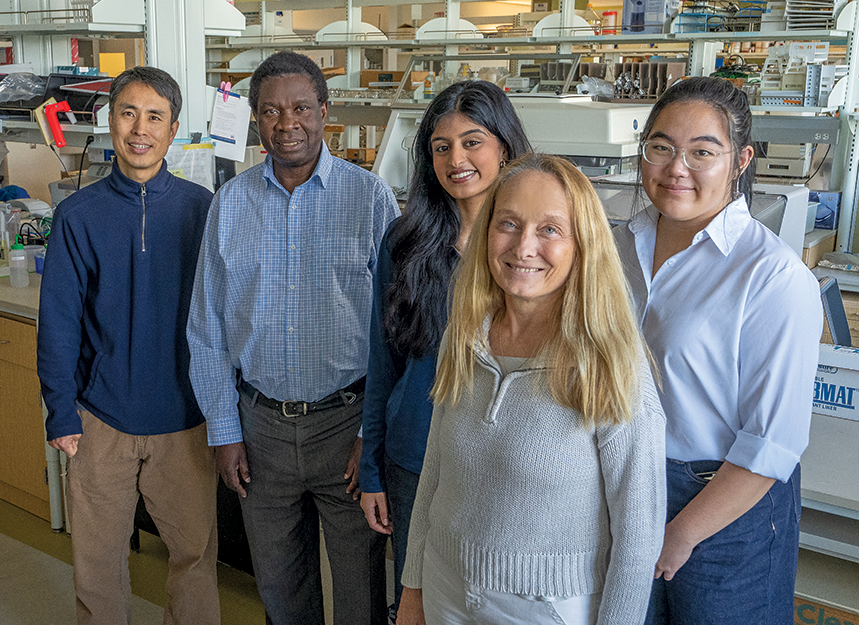
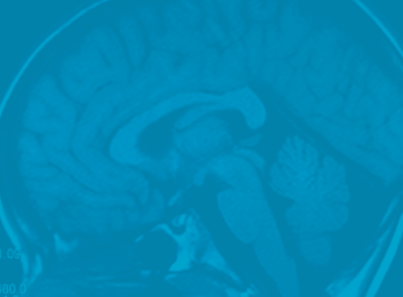
Damage to the nervous system, especially the brain, can result from several factors, including toxic chemicals such as hydrogen sulfide.
Neurodegeneration—or damage to the nervous system, especially the brain—can result from several factors, including toxic chemicals such as hydrogen sulfide (H2S). Novel research led by Professors Wilson K. Rumbeiha and Cecilia Giulivi has unlocked the secret to how this poisonous gas disrupts mitochondrial function, causing neurological damage.
Hydrogen sulfide is produced as a neurotransmitter at low levels by all mammals and appears to have a role in reducing inflammation. In spite of its usefulness, it can also act as a neurotoxin, and it’s the dose that makes the poison.
In the environment, H2S is a natural byproduct of decaying organic matter with a characteristic rotting egg odor. It is also a toxic byproduct of sewage, farming, wastewater treatment, and oil and gas industries. Effects of acute H2S poisoning include seizures and respiratory depression, followed by coma and death.
The team’s recent publication in Scientific Reports outlines how the compound caused changes to mitochondrial morphology that are likely to contribute to its neurodegenerative properties. They hope their investigation will lead to treatments for acute H2S exposure.
Mitochondria are powerhouses of the cell. They produce adenosine triphosphate (ATP), a molecule that fuels cellular processes. The brain requires a lot of energy, so malfunctioning mitochondria can affect executive and cognitive functioning. Past research has shown that toxic doses of H2S can damage brain mitochondria; however, the physical effects of H2S on mitochondrial morphology were unknown until the UC Davis researchers began their investigations.
The Department of Homeland Security is interested in hydrogen sulfide because it’s a very acute toxin, just like cyanide. There’s a need to understand how it affects the brain so we can develop antidotes and diagnostic tests in case of a mass civilian exposure.”
—Professor Wilson Rumbeiha
Hydrogen sulfide is a toxic byproduct of sewage, farming, wastewater treatment, and oil and gas industries.
“The Department of Homeland Security is interested in hydrogen sulfide because it’s a very acute toxin, just like cyanide,” said Rumbeiha. “There’s a need to understand how it affects the brain so we can develop antidotes and diagnostic tests in case of a mass civilian exposure.”
Rumbeiha, a veterinary toxicologist, in collaboration with Giulivi’s team, used a mouse model to mimic human exposure to large accidental or intentional exposure to H2S and assessed how mitochondrial changes in the brain occurred over time. They found distinct changes to the mitochondria in the mice’s thalamus.
“Changes happen as early as 12 hours post-exposure when you begin to see some disrupted morphology of mitochondria,” said Rumbeiha. “There was also damage to the cristae, the membrane where ATP is synthesized. Our teams describe a detailed landscape of morphological changes never reported before.”
Mitochondria are usually able to replicate a healthy copy through fission. “We found that H2S exposure led to dumbbellshaped mitochondria that were not able to completely divide and separate,” said Giulivi, who specializes in mitochondrial biology in neurological diseases. “Abnormal fusion of damaged mitochondria (not processed for clearance) can also explain these anomalies.”
Damage to mitochondria is linked to many disorders, including neurodegeneration. Ultimately, this work will lead to discovery of treatments for H2S exposure by warding off mitochondrial damage.
The researchers were also interested in establishing safe levels of H2S exposure. Currently, workers can be exposed to up to 10 ppm H2S, a level that Rumbeiha has shown compromises the immune systems of mice. Similar levels may affect human immune systems.
He also sees this research as an avenue to better understand the development of other neurodegenerative brain disorders.
“Neurodegenerative diseases like Alzheimer’s and Parkinson’s are a big problem in this country,” he said. “This type of research could offer us clues that lead to a better understanding of mechanisms and treatment of environmentally-induced neurodegeneration.” —By Jen DeMoss
5. Revealing Common Parasite’s Secret to Success
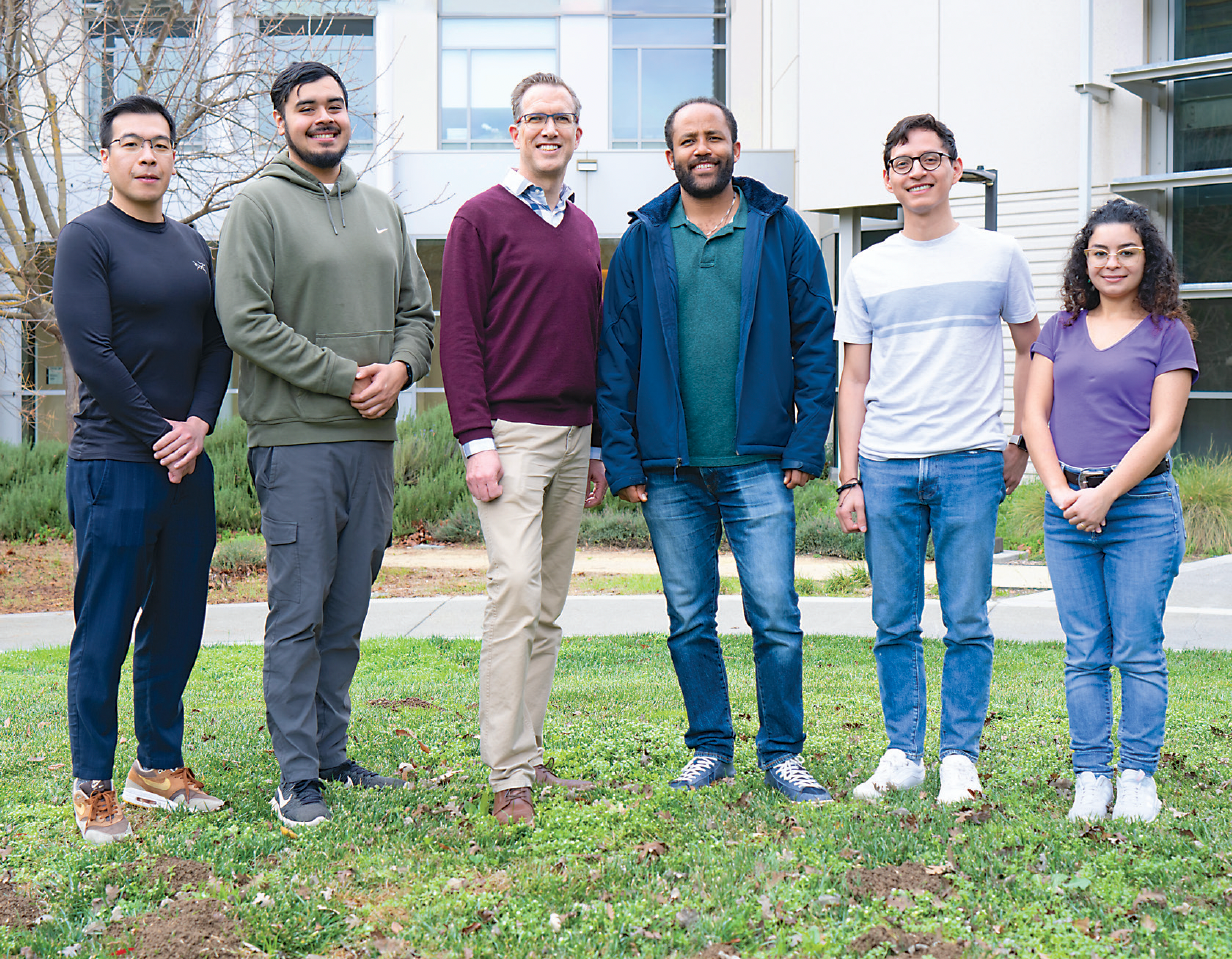
Professor Saeij explained that his team’s work focused on proteins like those produced by plant seeds to protect the embryos from drought and cold temperatures.
Toxoplasma gondii is a widespread, highly infectious parasite that can cause significant reproductive harm to humans, livestock, and wildlife. Recent publications by UC Davis veterinary medicine researchers outline gene discoveries that give T. gondii a remarkable resistance to environmental insults and the human immune system. Their work is paving the way for improved vaccines and further research to reduce the health effects of this common parasite.
A single-celled protozoan widely known to cause spontaneous abortion and death in livestock, T. gondii can cause miscarriages, neonatal death, birth defects, and severe health problems in humans, with immunocompromised individuals at particularly high risk.
Fertilized T. gondii eggs, called oocysts, are shed in the feces of cats, the parasite’s reproductive host. When other animals ingest the eggs, T. gondii sets up shop inside muscle and brain tissue. Humans can become infected from eating undercooked meat or handling waste from cat litter boxes.
“When a healthy person is infected for the first time, T. gondii replicates and disseminates throughout their body, eventually forming a lifelong chronic infection in their brain,” said Professor and senior author Jeroen Saeij. “If you're immunocompromised, an infection can reactivate and cause severe neurological problems.”
A single-celled protozoan widely known to cause spontaneous abortion and death in livestock, T. gondii can cause miscarriages, neonatal death, birth defects, and severe health problems in humans, with immunocompromised individuals at particularly high risk.
The secret to the protozoan’s success is the oocysts’ resistance to environmental stressors, including desiccation, freezethaw cycles, and high salinity. They can lay dormant for years, and then come alive as soon as they are ingested. A new study by Saeij and colleagues in the journal mBio describes the factors that contribute to the oocysts’ resilience.
Saeij explained that his team’s work focused on proteins like those produced by plant seeds to protect the embryos from drought and cold temperatures. The researchers knocked out four genes in the parasite’s genome that produce the proteins and compared the modified oocysts to wild T. gondii oocysts. The modified oocysts did not survive challenging environmental conditions nearly as well as wild T. gondii.
Their experiments have implications for vaccine development. Live T. gondii vaccines, currently only approved for use in sheep, must be stored at low temperatures and used soon after production, which can be a barrier to widespread use. Saeij thinks the proteins could be expressed in the vaccine strain to better preserve this or other live vaccines.
In a second mBio publication, Saeij and his research team explored which genes allow T. gondii to evade the human immune system. During an infection, immune cells release the protein interferon gamma, which stimulates surrounding cells to kill pathogens. While most T. gondii parasites perish due to interferon gamma, some escape to continue the infection.
In a painstaking process, Saeij’s team used CRISPR gene editing tools to knock out every gene in the T. gondii genome. They were left with a pool of modified parasites, each missing one gene, which they grew in cells that were either stimulated with interferon gamma or unstimulated. Parasites lacking genes to protect them from the interferon gamma environment perished in the stimulated cells.
“We’re not entirely sure yet why these genes are important to T. gondii,” said Saeij. “But this paper provides the first genome-wide overview of the ones that are specifically involved in resisting the interferon gamma response and offers years of further scientific exploration.” —By Jen DeMoss
6. Strengthening Our Bones
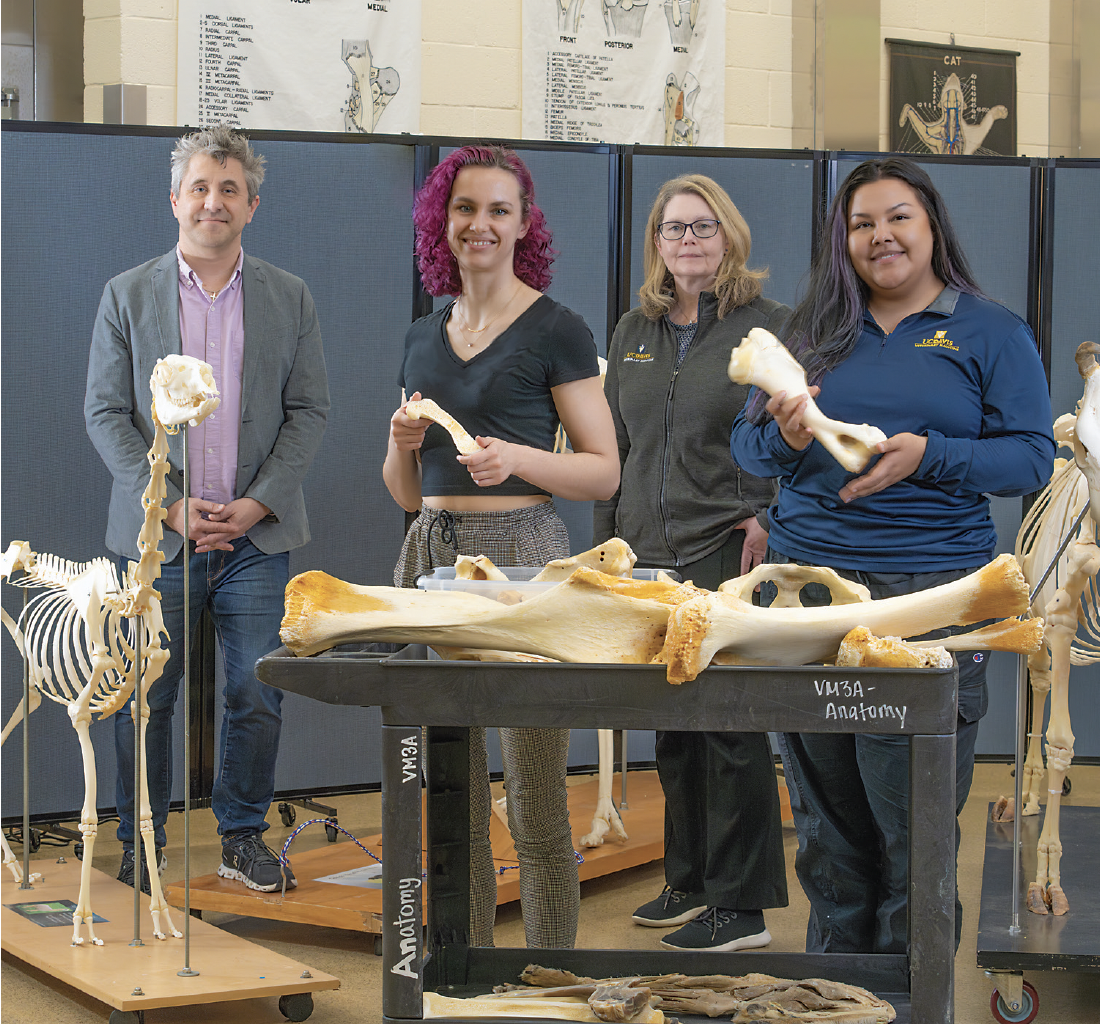
A new molecular target in a mouse model for novel therapies may increase bone mass and strength.
As we age, we lose bone mass, and the skeletal structure can become more weak and brittle, increasing fracture risk. This process, known as osteoporosis, is the major cause of fractures in postmenopausal women and older men. Current treatments have limited efficacy, leaving a demand for improved therapies to build bone and decrease fracture risk.
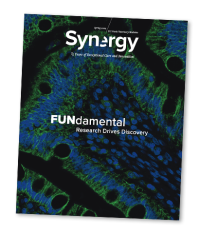
Foundational research may help develop better treatments. In a study recently published in Journal of Bone and Mineral Research Plus (and featured on this issue's cover), Professors Damian Genetos and Clare Yellowley, along with graduate student Sarah Mendoza, identified a new molecular target in a mouse model for novel therapies that may increase bone mass and strength.
They found that deletion of von Hippel-Lindau tumor suppressor protein (Vhl) in osteocytes— the most abundant cells in bone—produced a striking increase in bone mass. Vhl regulates the destruction of low oxygen sensing proteins known as hypoxia inducible factoralpha (HIF-α) subunits.
These HIF proteins allow osteocytes to respond to reductions in blood supply and oxygen availability to bone, as well as at fracture sites where blood vessels are broken. By deleting Vhl, the researchers were able to prevent the destruction of HIFs and prolong their ability to signal osteocytes to build bone.
“Bone is very dynamic, mechanically active tissue,” Genetos said. “It’s constantly remodeling, tearing down and building it back up to repair little cracks before they become traumatic fractures.”
Early therapies for osteoporosis were focused on stopping bone loss, which works, to an extent. The problem is that some of those drugs are too efficient and they prevent remodeling, so it actually increases fracture risk, Yellowley explained. Another issue with current osteoporosis therapies is that they are for limited time use and as soon as a patient stops taking the drugs, they slowly start losing bone mass.
“We need to progress from stopping bone resorption to increasing bone formation,” she said. “If we can identify molecular pathways that we can turn on or off that result in a high bone mass, we can potentially target those pathways with drugs to turn them on or off.”
This study culminates from 15 years of work that started with a small National Institutes of Health grant and a bit of serendipity. Genetos had been interested in HIF transcription factors, but most researchers were looking at their importance in osteoblasts—cells that build bone. However, osteoblasts don’t live long. They do their job and die or get trapped and become different cells—osteocytes—that can live for 30-50 years and help control bone formation and resorption.
So Genetos began looking at the impact of HIF proteins in osteocytes versus osteoblasts. That research led to current studies focused on known and novel molecular pathways that might be involved in Vhl-associated bone accumulation. Their goal is to understand how manipulation of these pathways affect the skeleton for the development of novel bone-regenerative therapies.
“Science has done a good job of extending our lifespan but the health span extension doesn’t match yet,” Yellowley said. “Ultimately, we hope this research will enable new therapeutics that will promote bone formation and increase mobility and quality of life in all people as they age.” —By Trina Wood